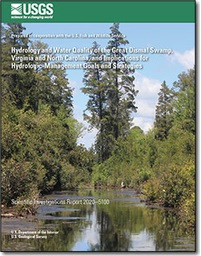
Product Details
- Product Number
- 534320
- Series
- SIR 2020-5100
- Scale
- NO SCALE
- Alternate ID
- SIR-2020-5100
- ISBN
- 978-1-4113-4518-8
- Authors
- FREDERIC C WURSTER
- Version Date
- 01/01/2020
- Regions
- NC, VA
- Countries
- USA
- Media
- Paper
- Format
- Bound
Additional Details
- Description
-
Hydrology and Water Quality of the Great Dismal Swamp, Virginia and North Carolina, and Implications for Hydrologic-Management Goals and Strategies
Scientific Investigations Report 2020-5100
Prepared in cooperation with the U.S. Fish and Wildlife Service
By: Gary K. Speiran and Frederic C. Wurster
https://doi.org/10.3133/sir20205100
First posted October 21, 2021
For additional information, contact:
Center Director,
dc_va@usgs.gov
Virginia and West Virginia Water Science Center
https://www.usgs.gov/centers/va-wv-water
U.S. Geological Survey
1730 East Parham Road
Richmond, VA 23228
Abstract
The Great Dismal Swamp is a peat wetland in the Coastal Plain of southeastern Virginia and northeastern North Carolina. Timber harvesting and the construction of ditches to drain the swamp and facilitate the harvesting are collectively implicated in changes that altered the wetland forests, caused subsidence and decomposition of the peat, and increased the risk of fire. In response to these changes, managers have implemented strategies to control water levels and rewet the swamp using a network of 64 adjustable-height, water-control structures on the ditches. Rewetting the swamp is intended to re-establish the original wetland-forest types, reduce the risk of fire, reduce subsidence and decomposition of the peat, enhance peat accretion, and reduce the risk of fire. Knowledge of responses of the swamp to hydrologic controls, however, is critical to developing and implementing effective management goals and strategies. Because the 2008 South One fire reemphasized the need for this knowledge, the U.S. Geological Survey in cooperation with the U.S. Fish and Wildlife Service began studies in 2009 to identify critical hydrologic controls and responses to these controls.
These studies identified water sources, topography, the two-layered hydraulic characteristics of the peat, the absence of peat in some areas, the ditch and road network, water-control structures on the ditches, the Dismal Swamp Canal and associated infrastructure, and wetland forests as the primary hydrologic controls. Precipitation is the only water source across much of the swamp. The eastward flow of streams and groundwater from the Isle of Wight Plain, across the Suffolk scarp, and into the swamp are additional water sources to the western part of the swamp. Vertical differences in the hydraulic characteristics of the peat reflect an upper peat having a high hydraulic conductivity and specific yield overlying a lower peat and sand having lower hydraulic conductivity and specific yield. The upper peat forms the main aquifer for the storage, flow, and release of water from the swamp. Maintaining water in the upper peat is critical to water availability to the wetland forests because of these properties.
Groundwater flows from the swamp into the ditches and the Dismal Swamp Canal where it discharges into nearby streams. Discharge typically is to the closest ditch except where a spoil-pile road that impedes flow intervenes between the swamp and the ditch. When groundwater levels in a ditch are about 2 feet lower than levels in the other three ditches surrounding a part of the swamp, however, most groundwater typically discharges to the ditch having the lower level. This occurs even if a spoil-pile road intervenes between the swamp and the ditch having the lower level. Flow to a single ditch shifts watershed boundaries and groundwater divides toward the ditches having higher water levels and demonstrates how flow and discharge are controlled by ditch water levels. Consequently, managing water levels based on these and other hydrologic controls and responses is critical to achieving management objectives.
The chemistry of water across the swamp shows the effects of the peat. Dissolved organic carbon concentrations in the groundwater are among the highest reported globally, ranging from 55 to 195 milligrams per liter. The pH of groundwater and ditch water is commonly less than 4.0 standard units because of organic acids. A relation between the pH and specific conductance of groundwater and ditch water reflects water sources, flow paths, and the chemical evolution, as waters from the different sources mix and flow along the paths.
- Print Date
- 2020
- Height In Inches
- 11.000
- Length In Inches
- 8.500
- Two Sided
- Yes
- Pieces
- 1
- Languages
- English