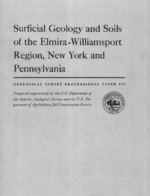
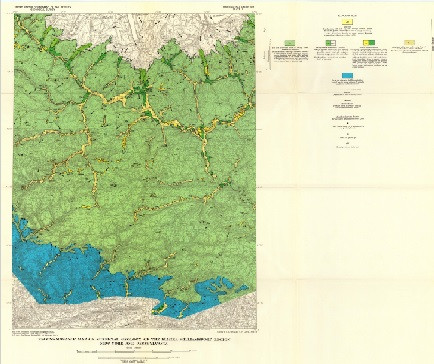
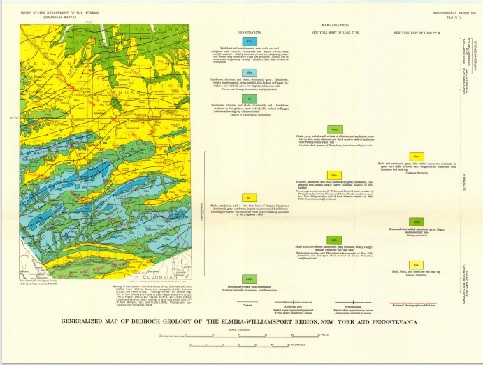
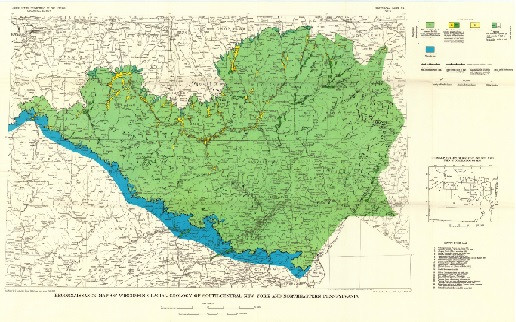
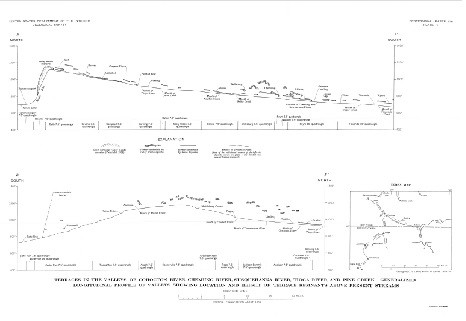
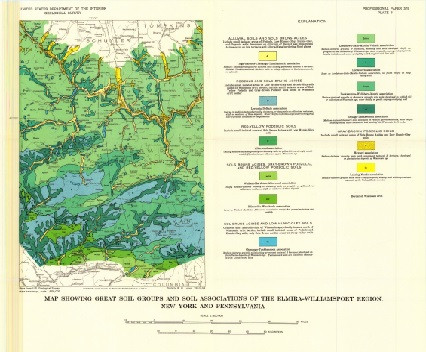
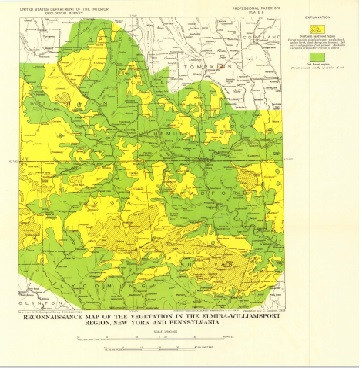
ELMIRA-WILLIAMSPORT REGION, NY & PA
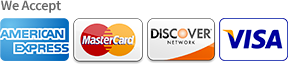
Product Details
- Product Number
- 11691
- Series
- PP-379
- Scale
- NO SCALE
- Alternate ID
- 16-0379
- ISBN
- 978-0-607-69794-0
- Authors
- LYFORD, WALTER HENRY
- Version Date
- 01/01/1963
- Regions
- NV
- Countries
- USA
- Media
- Paper
- Format
- Bound
Additional Details
- Description
-
Surficial geology and soils of the Elmira-Williamsport region, New York and Pennsylvania, with a section on forest regions and great soil groups
Professional Paper 379
By: Charles Storrow Denny, Walter Henry Lyford, and J. C. Goodlett
https://doi.org/10.3133/pp379
Abstract
The Elmira-Williamsport region, lying south of the Finger Lakes in central New York and northern Pennsylvania, is part of the Appalachian Plateaus physiographic province. A small segment of the Valley and Ridge province is included near the south border. In 1953 and 1954, the authors, a geologist and a soil scientist, made a reconnaissance of about 5,000 square miles extending southward from the Finger Lakes, N.Y., to Williamsport, Pa., and eastward from Wellsboro, Pa., to Towanda, Pa. Glacial drift of Wisconsin age, covering the central and most of the northern parts of the region, belongs to the Olean substage of MacClintock and Apfel. This drift is thin and patchy, is composed of the relatively soft sandstones, siltstone, shales, and conglomerates of the plateaus, commonly has a low calcium carbonate content, and is deeply leached. Mantling its surface are extensive rubbly colluvial deposits. No conspicuous terminal moraine marks the relatively straight border of Olean drift. The Valley Heads moraine of Fairchild near the south ends of the Finger Lakes is composed of relatively thick drift containing a considerable amount of somewhat resistant sedimentary and crystalline rocks. Commonly this drift has a relatively high carbonate content and is leached to only shallow depths. The Valley Heads drift is younger than Olean, but its precise age is undetermined. The age of the Olean is perhaps between Sangamon and Farmdale, on the basis of, in part, a carbon-14 date from peat at Otto, N.Y. All differences in soil development on these two Wisconsin drifts are clearly related to the lithology of the parent material or the drainage, rather than to weathering differing in kind or in duration. The authors believe that the soils are relatively young, are in equilibrium with the present environment, and contain few, if any, features acquired during past weathering intervals. The effect of tree throw on soil profiles and the presence of soils on slopes clearly indicate that soils form rapidly. Sols Bruns Acides are the most extensive great soil group occurring throughout the region. Podzols and Gray-Brown Podzolic soils are also widespread, and on long, smooth slopes Low Humic-Gley soils are common. Organic soils are of small extent. South of the Wisconsin drift border, the surficial mantle consists chiefly of alluvial, colluvial, or residual deposits of Wisconsin or of Recent age, but there are many small isolated patches of older, strongly weathered materials of pre-Wisconsin age. Although such older materials are commonly overlain or mixed with less weathered mantle, the yellowish-red color, characteristic of the strongly weathered material, is generally not masked. Some of the older material is drift, presumed to be of Illionian age, that was probably strongly weathered to a considerable depth in Sangamon time and has been greatly eroded since the last interglacial period. No clear-cut exposure of Wisconsin drift resting on older drift or other strongly weathered mantle has been found. The old drift and the other strongly weathered materials apparently acquired their present red color in pre-Wisconsin time. Where exposed at the surface, such strongly weathered mantle is the parent material of modern Red-Yellow Podzolic soils. Sols Bruns Acides and Gray-Brown Podzolic soils, developed on slightly weathered parent materials, are found adjacent to these red soils. This suggests that these Red-Yellow Podzolic soils probably developed from strongly weathered parent materials. No buried soils were found nor were any soils recognized as relics from pre-Wisconsin time. Comparison of a map of the great soil groups with a map of the vegetation of the region, prepared by John C. Goodlett, does not reveal a close relation. Laboratory analyses of samples collected furnish data on textural, mineralogical, and chemical changes caused by weathering and soil formation. The results indicate that the amount of chemical weathering which the Wisconsin drift has undergone is slight. The Red-Yellow Podzolic soils on strongly weathered pre-Wisconsin drift have B2 horizons that have a finer texture than the A2 or C horizons. The parent materials of these soils seem to be strongly weathered because of the high chromas, reddish hues, friable condition of most rock fragments, relatively high kaolinite content, and presence of gibbsite in the clay fraction. Measurements at numerous localities show that the depth of leaching increases with decreasing carbonate content and is not a criterion of the age of the drift. Pebble counts of gravels also show that the depth of leaching of gravel is related to its limestone content. The location of the gravel deposits is probably due primarily to the presence of pebbles of resistant rock rather than to ice wastage involving abundant glacial melt water. The region is in the Susquehanna drainage basin except for its north fringe, which drains to Lake Ontario. Most of the region is a dissected plateau ranging in altitude from 700 to 2,500 feet and underlain by gently folded sedimentary rocks of Paleozoic age. Much of the region slopes moderately or steeply; the most extensive areas of gently sloping land are 011 the uplands. In the northern part are several straight and deep valleys the southern extension of the Finger Lakes basins separated by uplands with several low cuestas that face north. Similarly, some streams such as the Canisteo, Cohocton, and Chemung Rivers, and the part of the Susquehanna River that is in New York, trend at right angles to the Finger Lakes, flowing in valleys that parallel the regional strike of the bedrock. The Olean drift border is marked by a change from drift containing very few rounded or striated rock fragments to a mantle containing only angular rock fragments and traces of red, strongly weathered materials. A reconstruction of the surface of the ice sheet, at its maximum extent shows an inferred slope of its distal margin ranging from 100 to 500 feet per mile
- Two Sided
- No
- Pieces
- 1
- Languages
- English