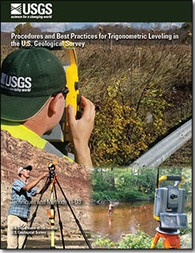
Product Details
- Product Number
- 534065
- Series
- TM-11-D03
- Scale
- NO SCALE
- Alternate ID
- TM-11-D03
- ISBN
- 978-1-4113-4443-3
- Authors
- MICHAEL L NOLL
- Version Date
- 01/01/2020
- Countries
- USA
- Media
- Paper
- Format
- Bound
Additional Details
- Description
-
Procedures and best practices for trigonometric leveling in the U.S. Geological Survey
First posted December 23, 2020
For additional information, contact:
Director, New York Water Science Center U.S. Geological Survey 425 Jordan Road Troy, NY 12180–8349
Abstract
With the advent of highly precise total stations and modern surveying instrumentation, trigonometric leveling has become a compelling alternative to conventional leveling methods for establishing vertical-control networks and for perpetuating a datum to field sites. Previous studies of trigonometric-leveling measurement uncertainty proclaim that first-, second-, and third-order accuracies may be achieved if strict leveling protocols are rigorously observed. Common field techniques to obtain quality results include averaging zenith angles and slope distances observed in direct and reverse instrument orientation (F1 and F2, respectively), multiple sets of reciprocal observations, quality meteorological observations to correct for the effects of atmospheric refraction, and electronic distance measurements that generally do not exceed 500 feet. In general, third-order specifications are required for differences between F1 and F2 zenith angles and slope distances; differences between redundant instrument-height measurements; section misclosure determined from reciprocal observations; and closure error for closed traverse. For F1 observations such as backsight check and check shots, the construction-grade specification is required for elevation differences between known and observed values.
Recommended specifications for trigonometric-leveling equipment include a total station instrument with an angular uncertainty specification less than or equal to plus or minus 5 arc-seconds equipped with an integrated electronic distance measurement device with an uncertainty specification of less than or equal to plus or minus 3 millimeters plus 3 parts per million. A paired data collector or integrated microprocessor should have the capability to average multiple sets of measurements in direct and reverse instrument orientation. Redundant and independent measurements by the survey crew and automated or manual reduction of slant heights to the vertical equivalent are recommended to obtain quality instrument heights. Horizontal and vertical collimation tests should be conducted daily during trigonometric-leveling surveys, and electronic distance-measurement instruments should be tested annually on calibrated baselines maintained by the National Geodetic Survey. Specifications that were developed by the National Geodetic Survey for geodetic leveling have been adapted by the U.S. Geological Survey (USGS) for the purpose of developing standards for trigonometric leveling, which are identified as USGS Trigonometric Level I (TL I), USGS Trigonometric Level II (TL II), USGS Trigonometric Level III (TL III), and USGS Trigonometric Level IV (TL IV). TL I, TL II, and TL III surveys have a combination of first, second, and third geodetic leveling specifications that have been modified for plane leveling. The TL III category also has specifications that are adapted from construction-grade standards, which are not recognized by the National Geodetic Survey for geodetic leveling. A TL IV survey represents a leveling approach that does not generally meet criteria of a TL I, TL II, or TL III survey.
Site conditions, such as highly variable topography, and the need for cost-effective, rapid, and accurate data collection in response to coastal or inland flooding have emphasized the need for an alternative approach to conventional leveling methods. Trigonometric leveling and the quality-assurance methods described in this manual will accommodate most site and environmental conditions, but measurement uncertainty is potentially variable and dependent on the survey method. Two types of closed traverse surveys have been identified as reliable methods to establish and perpetuate vertical control: the single-run loop traverse and double-run spur traverse. Leveling measurements for a double-run spur traverse are made in the forward direction from the origin to the destination and are then retraced along the same leveling route in the backward direction, from the destination to the origin. Every control point in a double-run spur traverse is occupied twice. Leveling measurements for a single-run loop traverse are made in the forward direction from the origin point to the destination, and then from the destination to the origin point, along a different leveling route. The only point that is redundantly occupied for the single-run loop traverse is the origin. An open traverse method is also considered an acceptable approach to establish and perpetuate vertical control if the foresight prism height is changed between measurement sets to ensure at least two independent observations. A modified version of leap-frog leveling is recommended for all traverse surveys because it reduces measurement uncertainty by forcing the surveying instrumentation into a level and centered condition over the ground point as the instrumentation is advanced to the objective. Sideshots are considered any radial measurement made from the total station that is not part of a traverse survey. F1 and F2 observations are recommended for sideshots measurements for projects that require precise elevations. Quality-assurance measurements made in F1 from the station to network-control points should be considered for surveys that require a high quantity of sideshots.
The accuracy of a trigonometric-leveling survey essentially depends on four components (1) the skill and experience of the surveyor, (2) the environmental or site conditions, (3) the surveying method, and (4) the quality of the surveying instrumentation. Although components one and two can sometimes be difficult to evaluate and be highly variable, the objective of this manual is to disseminate information needed to identify, maintain, and operate quality land-surveying instrumentation, and to document procedures and best practices for preparing and executing precision trigonometric-leveling surveys in the USGS.
- Survey Date
- 2020
- Print Date
- 2020
- Height In Inches
- 11.000
- Length In Inches
- 8.500
- Two Sided
- Yes
- Pieces
- 1